Experiments on fundamental nonlinear interactions
between Alfvén waves are being performed in the Large Plasma
Device (LAPD) at UCLA. Interactions between counterpropagating shear
waves are fundamental to the Alfvén cascade. The
focus of our studies is on: (1) individual wave-wave collisions
between counter-propagating waves and (2) the cumulative effects of
many wave-wave collisions, realized through ``stirring'' with a large
amplitude Alfvén wave in a cavity (reflecting boundary
conditions). We are studying these interactions for: anisotropy
(preference for cascade in the direction perpendicular to the field);
dependence on launch wave amplitude, frequency, and polarization; and
influence of non-ideal effects (coupling to compressible modes
(e.g., slow magnetosonic modes), kinetic and inertial shear wave
cascades). These experimental results will be used to test both
theories and simulations of the Alfvén cascade. In particular,
experiments in the LAPD are well suited to simulation by the GS2
electromagnetic code. We intend to provide a important experimental
benchmark for this code through detailed measurements of this
nonlinear process in LAPD.
Using LAPD typical parameters and assuming a perpendicular wavelength
of 30 centimeters for our outer scale wave, we find a constraint on
the largest launch frequency for the stirring wave in order to avoid
cyclotron damping before reaching Landau damping scales. Written as a
constraint on the parallel wavelength of the stirring wave:
λparallel > 32 meters. This is less than the
wavelength of the largest possible wave in LAPD, making a cascade over
two orders of magnitude in perpendicular scale possible in LAPD. The
constraint on the launch frequency is relaxed if the initial
perpendicular wavelength is decreased (e.g., if we consider
cascades over shorter distances in k space).
There are also requirements on the launch wave amplitude in order to
easily observe this nonlinear process in LAPD. If we want the cascade
to be strong, this requires that the outer scale wave amplitude
satisfy δB/B ~
kpar/kperp ~ 1%. This wave
amplitude has already been achieved in early experiments with
high-amplitude shear wave generation in LAPD. In the
strong cascade, the nonlinear eddy turnover time (time for the
nonlinear perturbation to grow to unity) is comparable to the period
of the interacting Alfvén waves. In this regime, nonlinear
perturbations arising from single wavepacket collisions will likely be
observable and can be studied. These interactions will be studied for
anisotropy (preference for structure formation in the perpendicular
direction) and dependence on launch wave parameters (frequency,
polarization). The strong cascade will be studied through establishing
reflecting boundary conditions in LAPD using the conducting
anode/cathode as one boundary and a metal plate as the second,
allowing multiple interactions between reflected counter-propagating
Alfvén waves. We will study the wavenmuber spectrum established by
the cascade (again, looking for anisotropy) and dissipation and
heating at skin depth or ion gyroradius scales. We will also look for
evidence of a continuation of the cascade in Whistler waves beyond
these scales (on the order of 0.5 centimeters in LAPD).
The development of large amplitude shear Alfvén wave drivers for
LAPD is central to our research program. Two techniques have now been
successfully used to launch shear waves with δB/B ~ 1%.
The first makes use of recent observation of an Alfvén wave
``maser'' in LAPD. Here an instability driven by inverse Landau
damping in the anode-cathode region has been observed to generate
large amplitude shear Alfvén waves in the source
cavity. This instability excites the fundamental
Alfvénic mode of the resonant cavity defined by the Nickel cathode
and the partially reflecting Molybdenum mesh anode, 0.5 meters away.
The figure above shows example traces of discharge current, signal
from a magnetic pick-up loop, and pickup-loop wavelet power spectrum
during a discharge where the instability is excited.
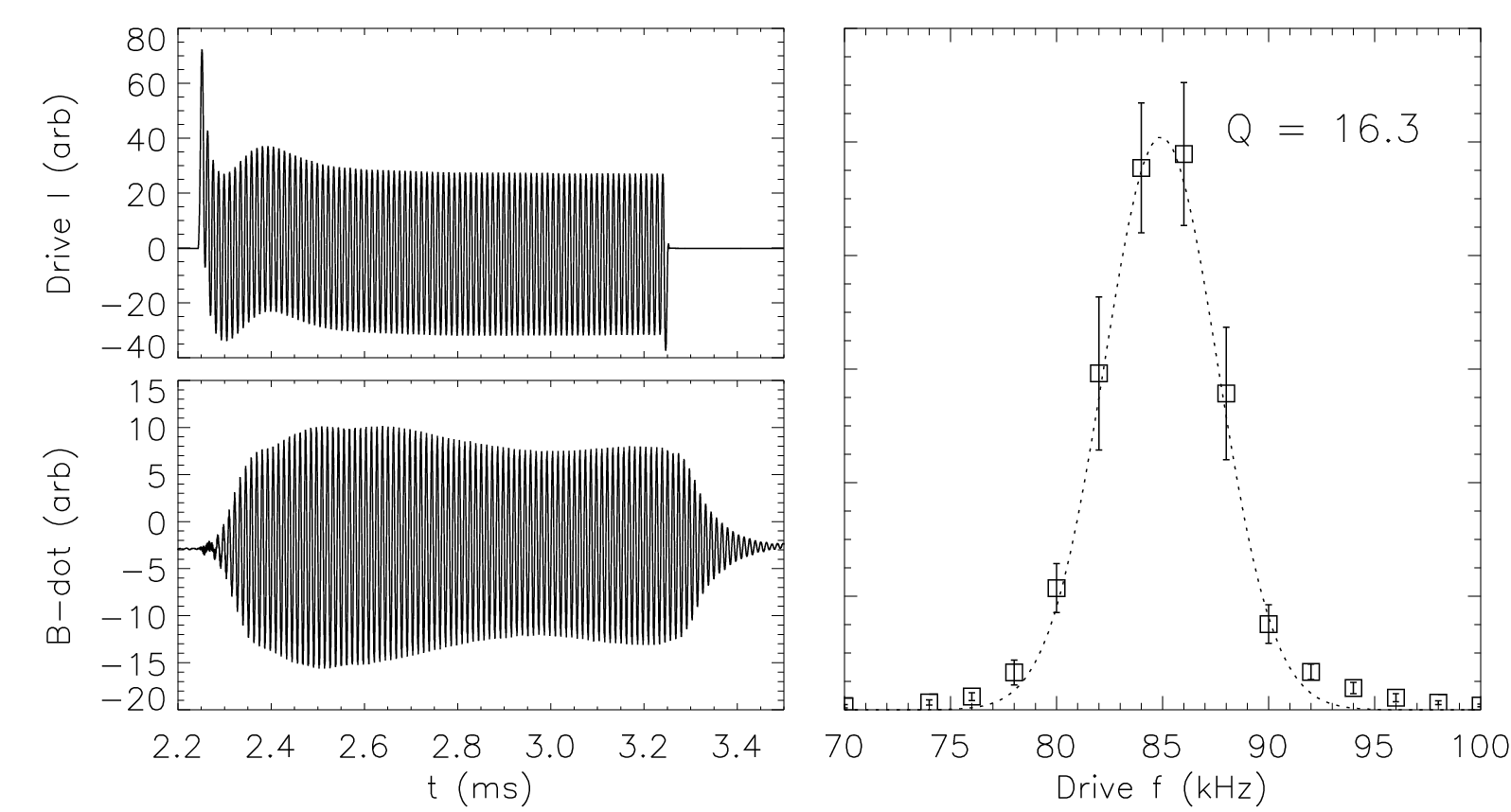
|
This resonant cavity can also be externally excited to generate
Alfvén waves. An initial test of this idea has been performed,
using a broadband power push-pull driving circuit which modulates the
discharge current in the anode-cathode region. This figure
shows the result of such an external excitation, including a
measurement of the Q of the anode-cathode cavity.
|
The second approach uses the same novel, broadband driver circuit to
drive a simple loop antenna (here the loop normal points perpendicular
to the field and the loop is rectangular, distended in the magnetic
field direction). It has recently been found that large amplitude
shear Alfvén waves (which are column eigenmodes) can be launched using
modest currents (100 A). Using this type of antenna, a broader range
of frequencies can be launched (i.e., not a resonant process).
The current antenna size (15 centimeters across the field by 31
centimeters along) is limited by available port size on the LAPD
vacuum vessel. Efficient coupling to lower frequency waves is
critical in order to easily create stirring waves in the strong
cascade regime and in order to maximize
the potential range in wavenumber space of a cascade in LAPD.
Development of new anode-cathode drivers (perhaps driving between a
cathode on one end of the machine and an anode at the other) or larger
loop antenna will be pursued to achieve creation of large amplitude,
low frequency Alfvén waves.
Initial studies of ``stirring'' with a large scale wave in reflecting
boundary conditions have been performed using the resonant
anode-cathode cavity as a wave source. Reflecting boundary conditions
are established by closing a conducting door internal to the LAPD
device, shortening the length of the machine to 10 meters. In these
initial studies, a strong nonlinear interaction between the launch
shear wave and a low-frequency density fluctuation is
observed. This is likely brought
about by the presence of two frequencies in the shear wave spectrum
emitted by the anode-cathode cavity (usually this is transient, and is
thought to be due to evolution of the plasma profiles allowing two
eigenmodes of the anode-cathode cavity to exist
simultaneously).
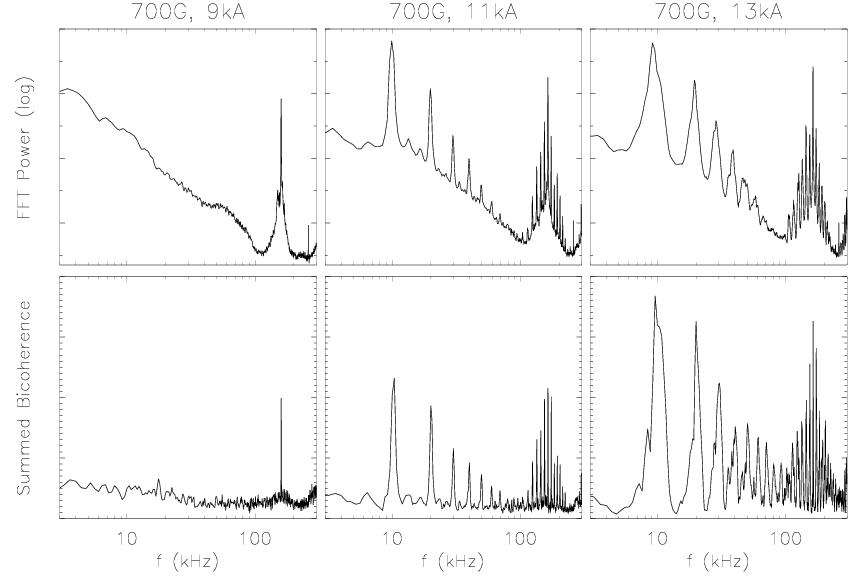
Spectrum and bicoherence (floating potential) during
experiments with reflecting boundary conditions |
This figure shows how the spectrum and
bicoherence of potential fluctuations changes with increasing Alfvén
wave amplitude (increasing discharge current). The experiments have
clearly already entered a nonlinearly relevant regime. As the
amplitude is increased (the amplitude of the MASER wave increases with
discharge current), sidebands develop around the original Alfvén
wave and a low frequency fluctuation develops at the sideband
separation frequency and harmonics. The bicoherence clearly indicates
three-wave interactions between the Alfvén waves and the low
frequency fluctuations. The low-frequency fluctuation is a propagating
wave in the plasma and is possibly an ion-acoustic or slow
magnetosonic wave created nonlinearly by the interaction of shear
waves differing in frequency by the ion acoustic frequency. This
possibility is currently being explored in new experimental
campaigns.
|
These experiments will allow detailed measurements of the nonlinear
physics of Alfvén wave interactions in the LAPD plasma. These
measurements can be used to provide stringent tests of theoretical
models and simulations in the LAPD parameter regime, providing more
confidence in the application of these to other problems (e.g.,
tokamak or astrophysical plasmas). A more detailed plan of work for
this project follows
|